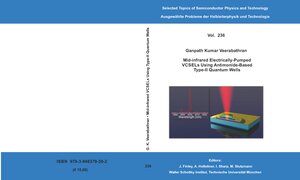
×
Mid-infrared Electrically-Pumped VCSELs Using Antimonide-Based Type-II Quantum Wells
von Ganpath Kumar VeerabathranThe mid-infrared (mid-IR) spectral region above 3 μm has a lot of potential for the detection of various gases such as CH4, NO2, SO2, CO2, O3 etc., which have strong absorption lines in this region. Highly sensitive optical gas sensing schemes like the tunable diode laser absorption spectroscopy (TDLAS) use this property to detect these gases. TDLAS requires continuous-wave (CW) electrically-pumped (EP) lasers with single-mode emission, mode-hop free wavelength tunability and low power consumption. Although there has been rapid progress on edge-emitting semiconductor lasers in the 2-6 μm range, their power consumption is typically a few hundreds of mWs making them unattractive for compact portable applications. Vertical-Cavity Surface-Emitting Lasers (VCSELs) on the other hand can easily satisfy the requirements of TDLAS for a fraction of the power consumption. Furthermore, they have other advantages such as larger electro-thermal tunability, smaller beam-divergence and low-cost potential. However, so far, the development of mid-IR VCSELs has been slow compared to their edge-emitting counterparts. Electrically pumped VCSELs emitting above 2.5 μm have only been achieved on the GaSb substrate. The main aim of this thesis is the development of a novel concept to extend the operating range of GaSb-based CW EP single-mode tunable VCSELs into the mid-IR region above 3 μm for gas sensing applications. The buried tunnel junction (BTJ) VCSEL concept has already yielded high-performance VCSELs between 1.5-3 μm. And type-II ’W’ quantum wells (QW) have been successfully used in cascaded configurations to make conventional edge-emitting lasers with excellent performances between 3-6 μm. Here, these two concepts have been combined to make a BTJ-VCSEL with a single-stage (non-cascaded) type-II active region and devices emitting at a record long wavelength of as much as 4 μm have been demonstrated.
Single-stage active regions emitting in the mid-IR using type-II QWs are uncommon in edge-emitting lasers due to the obvious advantages of cascading. However, cascading gain layers in GaSb-based VCSELs is very challenging due to the lack of a suitable concept for lateral current confinement. Therefore, in this work significant emphasis has been laid on the development of a suitable single-stage active region that would provide enough gain for a VCSEL. Properties of the developed active region such as its gain, transparency current density and temperature sensitivity, characterized using edge-emitting lasers, are discussed in detail. Following this, the design, fabrication and characterization of mid-IR VCSELs using the developed active region are presented. The low optical gain provided by type-II QWs coupled with the fact that optical absorption loss increases with the square of the wavelength demands a low optical loss cavity design for the VCSELs. Several design principles aimed at minimizing optical losses in mid-IR VCSELs are covered in this thesis.
The VCSELs described in this thesis comprise 8 type-II QWs in a single stage configuration between an undoped epitaxial bottom distributed Bragg reflector (DBR) and a dielectric top DBR. Each of the QWs is made of a GaInSb hole confining layer sandwiched between two InAs electron confining layers, with a squared electron-hole wavefunction overlap of 30%. The bottom mirror is made of 30-pairs of undoped epitaxially grown AlAsSb and GaSb, while the top mirror is made of 5-pairs of evaporated Ge and ZnS. A structured buried tunnel junction is used for current confinement and transverse waveguiding, while an intra-cavity contacting scheme is used for current injection. The 3.5-λ-thick optical cavity consists mostly of n-GaSb with modulated doping. An optimized doping profile is developed for the cavity that minimizes optical and ohmic losses. The realized devices operate in the CW mode up to 0°C and in the pulsed mode up to 45°C. Threshold pump powers of less than 10 mW are demonstrated in CW operation at temperatures easily accessible with thermo-electric cooling. They emit a maximum single-mode CW optical power of 180 μW at =40°C which is already sufficient for gas sensing applications. They exhibit a continuous electro-thermal wavelength tuning range of 19.2 nm, while operating in single-spectral mode over the entire tuning range with a side-mode suppression-ratio of more than 20 dB.
The devices described in this thesis are the first EP VCSELs in the world to emit at 4 μm, demonstrating the potential of the BTJ VCSEL technology. As the performance of the type-II active regions continuously degrades with increasing wavelength, the realization of the 4 μm devices indicates that it is feasible to achieve BTJ VCSELs within the entire 3-4 μm wavelength with the presented approach with at least comparable lasing performance. It seems that the major issue impeding CW operation at room temperature and higher output powers is the high threshold of the devices. Low gain and poor temperature stability of the type-II QWs and large lateral diffusion of charge carriers in the active region result in high thresholds. Therefore, further improvement in these devices should focus on reducing the thresholds. It is most promising to increase the squared wavefunction overlap of the QWs by increasing the indium content in the GaInSb hole-confining layer, which should significantly improve gain. An innovative concept to cascade such type-II QWs in BTJ VCSELs should tremendously improve their performances.