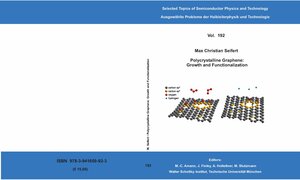
×
Polycrystalline Graphene: Growth and Functionalization
von Max Christian SeifertGraphene; a one-atom-thick planar sheet of carbon is seen as one of the most promising material systems for wearable and flexible electronics as well as for biosensing applications. In the framework of this thesis we aim to facilitate the industrial use of graphene and to advance the use of graphene in the field of sensing and biosensing applications.
In a first step we study the chemical vapor deposition (CVD) growth for the synthesis of high quality graphene on a large scale. We develop a time and cost-efficient growth technique based on the inductive heating of millimeter-thick metallic substrates. Here, the inductive heating unit enables fast temperature ramps while an electrochemical transfer procedure allows for the reuse of the costly growth substrates. Given the polycrystalline nature of CVD graphene, we optimize growth targeting a maximized size and quality of the individual single-crystalline grains and thus optimize the electronic performance of the grown graphene sheets.
In a second step, we focus on the controlled covalent modification of the graphene surface with hydrogen and oxygen. We successfully establish hydrogenation and ozonization methods that allow to precisely tune the density of the induced defect sites, as well as to tune the predominant defect type from surface-tethered sp3-hybrids to vacancies. We find that defects accumulate at the graphene grain boundaries for ozonized samples whereas graphene hydrogenation yields even defect distribution. Comparing electronic measurements of graphene field-effect transistors undergoing gradual oxidation/hydrogenation and quantum transport simulations, we are able to explain the observed degradation of the device mobility with the characteristics of the induced defects, in particular, their spatial distribution.
In a last step, against the background of analyte-specific sensing applications, we introduce the covalent grafting of polymer brushes on graphene as a non-invasive route towards graphene-based multifunctional sensing. We demonstrate that the controlled hydrogenation of graphene prior to polymerization can be used to tune the grafting density of polystyrene brushes. Finally, we develop copolymerization techniques that overcome the limitation of successful polymerization to styrene- based monomers and enable for the direct grafting of functional polymer brushes on graphene.
In a first step we study the chemical vapor deposition (CVD) growth for the synthesis of high quality graphene on a large scale. We develop a time and cost-efficient growth technique based on the inductive heating of millimeter-thick metallic substrates. Here, the inductive heating unit enables fast temperature ramps while an electrochemical transfer procedure allows for the reuse of the costly growth substrates. Given the polycrystalline nature of CVD graphene, we optimize growth targeting a maximized size and quality of the individual single-crystalline grains and thus optimize the electronic performance of the grown graphene sheets.
In a second step, we focus on the controlled covalent modification of the graphene surface with hydrogen and oxygen. We successfully establish hydrogenation and ozonization methods that allow to precisely tune the density of the induced defect sites, as well as to tune the predominant defect type from surface-tethered sp3-hybrids to vacancies. We find that defects accumulate at the graphene grain boundaries for ozonized samples whereas graphene hydrogenation yields even defect distribution. Comparing electronic measurements of graphene field-effect transistors undergoing gradual oxidation/hydrogenation and quantum transport simulations, we are able to explain the observed degradation of the device mobility with the characteristics of the induced defects, in particular, their spatial distribution.
In a last step, against the background of analyte-specific sensing applications, we introduce the covalent grafting of polymer brushes on graphene as a non-invasive route towards graphene-based multifunctional sensing. We demonstrate that the controlled hydrogenation of graphene prior to polymerization can be used to tune the grafting density of polystyrene brushes. Finally, we develop copolymerization techniques that overcome the limitation of successful polymerization to styrene- based monomers and enable for the direct grafting of functional polymer brushes on graphene.